In this article, we explore the various differences between different types of transformers and their equivalent circuits. Transformers are recognized as fundamental tools in the field of electricity, transferring electrical energy from one circuit to another. These electromagnetic devices operate based on the principles of magnetic field induction around a core, converting electrical currents. We delve into various types of transformers such as power transformers, current transformers, and telecommunications transformers, while also examining their equivalent circuits.
What is a Transformer?
A transformer is an electrical device that transfers electrical energy from one point to another. It is used in power grids and industries to increase or decrease voltage, current, and electrical power.
The function of a transformer is based on magnetic induction between coils, facilitating the transfer of energy from the primary coil to the secondary coil. During this process, the type and amount of energy remain unchanged, but voltage and current vary. The operational principles of transformers rely on mutual induction in the coils.
Transformer Structure
A transformer’s structure consists of two coils of wire and a magnetic core: the primary coil and the secondary coil. The primary coil transfers the magnetic field to the core, inducing a magnetic flux which then creates a current in the secondary coil. The secondary coil, in turn, is another type of winding that converts the changes in magnetic current to electrical current.
The transformer core is designed as a magnetic circuit with minimal air gap and low magnetic resistance to facilitate easy magnetic flux flow. The core structure is typically built laminated, with each lamination approximately 0.3 mm thick. Overall, the transformer core facilitates the transfer of voltage from the primary coil to the secondary coil. Without a core, due to magnetic dispersion, inducing voltage would not be possible.
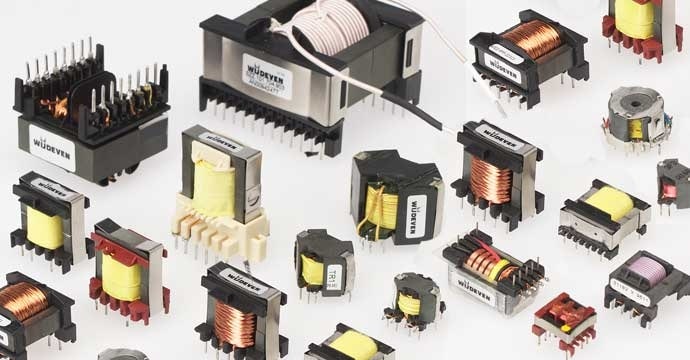
Types and Components of Transformers
Transformers can be broadly classified into two main types: step-up and step-down transformers. A transformer is classified as a step-up transformer if the number of turns in the secondary coil is greater than the primary coil, resulting in increased output voltage. Conversely, a step-down transformer decreases the output voltage compared to the input voltage.
Components of a Transformer
1. Core: As the heart of a transformer, the core is responsible for transferring the magnetic field and converting energy.
2. Windings: The primary and secondary windings respectively transfer magnetic flux from the source to the core and from the core to the load.
3. Oil Tank: Essential for cooling and insulation, the oil tank is a critical component in transformers.
4. Radiator: Used to enhance heat dissipation from the oil tank.
5. High and Low Voltage Bushings: These components connect the windings to transmission lines and perform electrical operations.
6. Tap Changer and Mechanism Panel: Used to adjust voltage and current and make changes within specified ranges.
7. Control Panel: Manages transformer operations and interfaces with the power system.
8. Measurement and Protection Devices: Include sensors and necessary tools for measuring and monitoring transformer parameters.
9. Valves and Connecting Pipes: Used to connect and facilitate communication between various transformer components.
10. Cooling Devices: Such as pumps and cooling systems to maintain optimal temperature within the transformer.
11. Current Transformer: Used for current transmission without voltage alteration.
12. Chassis and Wheels: Structural components for installation and transportation of the transformer.
Transformer Operation is Based on Two Fundamental Principles:
1. Electromagnetic Induction: Alternating electric current induces a variable magnetic field in the transformer core. This magnetic field induces an alternating current in the secondary winding wrapped around a core loop.
2. Transformer as an Energy Converter: This device transfers electrical energy from one circuit to another. In general operation, the primary and secondary windings are connected through a core with different voltages. If the voltage in the primary winding increases, this change is transmitted to the secondary winding, inducing current flow in it.
Read more: Types of Transformers and Their Equivalent Circuits
The Ideal Transformer: Efficient Energy Transfer and Functionality
An ideal transformer is a crucial device in the distribution and transmission of electrical energy, effectively transferring energy from one level to another. In this transformer, magnetic flux from the primary coil induces electric current in both the primary and secondary coils.
This functionality of the transformer is achieved through key components such as the core, windings, oil tank, radiator, high and low voltage bushings, tap changer and mechanism panel, control panel, measurement and protection devices, valves and connecting pipes, cooling devices, current transformer, and chassis and wheels. These components work together in harmony to facilitate the transformer’s operation and enable the transfer of electrical energy.
The primary feature of an ideal transformer is the use of a core structure designed to minimize magnetic dispersion losses. These cores enhance energy transfer efficiency and reduce losses due to magnetic dispersion.
Utilizing this technology, ideal transformers serve as stable energy sources for various systems. They are highly efficient and economically beneficial, playing a significant role in energy transmission with minimal losses.
To prevent power loss in ideal transformers, careful attention to voltage regulation and phase differences is essential. Additionally, the use of advanced technologies in the design and construction of these devices contributes to improved performance and reduced losses.
Ideal Transformers in Various Applications
Ideal transformers are utilized in applications where minimizing losses is crucial, employing identity methods such as variable magnetic flux methods for efficient energy transfer. These transformers play a vital role in equipment like welders, mercury vapor lamps, and neon signs to ensure safety, particularly in loads prone to short circuits.
The equivalent circuit of a transformer is a fundamental component in the field of electricity, aiding in the analysis and breakdown of transformer functionality. This circuit, utilizing simple electrical circuits, depicts the effect of magnetic flux in the transformer core and assists in precise related calculations.
Key features of this circuit include the resistances RS and RP, representing series and parallel resistances in transformer windings, crucial in voltage and current transformations.
By analyzing the properties of leakage flux, this circuit illustrates induced components XP and XS, which are in series with the windings and significantly affect the electrical characteristics of the transformer.
In the equivalent circuit, iron losses are divided into two categories: eddy current losses (Foucault losses) and hysteresis losses, which impact transformer performance and efficiency improvement. Detailed analysis of these circuits is essential for electrical engineers and specialists to enhance transformer performance and reduce losses.
In transformers, losses are directly related to the square of the core flux, and these losses vary proportionally with input voltage. These losses can be modeled as a parallel resistance in the transformer circuit, known as RC resistance.
In constant frequency conditions, a core with limited permeability requires current to maintain magnetic flux. Changes in magnetic flux are in phase with variations in core currents. Despite core saturation, these branches play separate roles, with resistances RS and RP accompanying magnetic flux changes.
In sinusoidal sources, magnetic flux lags 90 degrees behind induced voltage, which can be considered in parallel with core iron losses using the inducing element XM, naming this magnetic branch the magnetizing branch.
When the secondary winding of a transformer is opened, the no-load current passing through the magnetizing branch is known as current I.
Resistances RS and XS on the secondary side must be transferred to the primary side. These resistances represent copper losses and leakage inductance on the secondary side and are placed in series with the secondary winding. This equivalent circuit is employed in the detailed analysis of transformers in various applications.
Types of Transformers
Transformers, categorized by their cores and usage types, have various forms. Regarding core type, two primary types of transformers exist:
1. Air-Core Transformers: These transformers have a core made of materials such as plastic or wood. They are utilized as simple solutions suitable for environments requiring higher insulation.
2. Oil-Core Transformers: In these transformers, the core is constructed from ferrous metals like iron or steel and filled internally with a specific oil. Due to their higher strength and stability against diverse conditions, these transformers are considered suitable options for industrial and power plant applications.
Additionally, transformers are divided into various types based on their applications. For example:
– Power Transformers: These transformers are used to convert voltage levels in power plants and high-voltage substations. They are also utilized in applications such as aluminum smelting processes in furnaces.
– Distribution Transformers: These are fundamental tools in electricity distribution networks used for distributing electrical energy in cities and factories. These transformers are typically installed in ground-mounted or pole-mounted substations and are responsible for creating the neutral point to detect ground faults and imbalance in phase loads.
– Current Transformers (CT): Another vital component of the power grid used for measuring current and protecting circuits against current surges or short circuits. These transformers generate measurable and analyzable signals, helping to protect the electrical system against various hazards.
– Potential Transformers (PT): Also crucial in power systems for measuring voltage and protecting against voltage fluctuations. These transformers provide observable voltage signals essential for controlling and monitoring voltage in electrical systems.
– Capacitive Voltage Transformers (CVT) or Capacitor Voltage Transformers: Used for voltage measurement and protection, operating based on the principle of capacitive voltage division. These transformers are typically employed in high-voltage lines for exchanging telecommunication signals and play a significant role in protecting and controlling electrical systems.
Transformer matching or Maching transformer is also used for differential protection by balancing primary and secondary currents. This type of transformer is employed precisely in protective circuits to safeguard equipment and enhance the efficiency of electrical systems.
Oil-filled transformers, one of the most common types, use oil as an insulating and cooling medium. Due to the excellent thermal and insulation properties of oil, these transformers are typically employed in power plants and large electrical networks.
Dry-type transformers use solid insulation materials instead of oil to avoid the need for oil insulation. These transformers are suitable for environments where oil maintenance is not feasible due to the absence of oil.
SF6 gas-insulated transformers utilize SF6 gas as an insulating medium. This gas is an excellent cooling and insulating agent and is commonly used in equipment requiring high-voltage insulation in compact spaces.
In terms of winding, single-phase transformers use one phase wire and one ground wire for energy transmission. Meanwhile, two-phase transformers use two phase wires and one ground wire. Three-phase transformers with three-phase winding are highly efficient and effective in large-scale energy transmission, making them suitable for power grids and power plants.
The diversity of winding connections in transformers is one of the fundamental considerations in the design and utilization of these critical electrical devices. Among the commonly used connections, the Star-Star (Yy) connection is employed for bidirectional power transmission and applications requiring voltage boost. The Star-Delta (Yd) connection is used to increase voltage and reduce current in distribution systems.
Additionally, the Delta-Delta (Dd) connection is suitable for high power transmission and high currents, while the Delta-Star (Dy) connection is used for voltage reduction and current increase in distribution systems. The Star-Star connection with a neutral balancing transformer (Yy(dt)) is also suitable for maintaining voltage balance in systems with variable load differences.
Optimization of power and enhancement of transformer performance are achieved through their parallel operation in energy distribution stations. This method combines multiple transformers in parallel to increase power capacity and improve efficiency. This parallel connection must be carefully established and meet specific conditions to ensure optimal performance and system stability.
Certain essential conditions must be met for parallel operation of transformers to create a stable and highly efficient system. These conditions include several critical factors that must be meticulously observed:
The first condition for paralleling transformers is that their rated voltages must be equal. The rated voltage of both primary and secondary windings should be identical to ensure uniform distribution of currents.
Furthermore, transformers should be matched in terms of type and model to achieve compatibility in voltage and current performance. Equivalence in impedance connections is also crucial for maintaining balance in currents.
Phase synchronization is another essential condition. Phases of transformers with identical names must be connected to each other to achieve synchronization in currents and prevent any operational disruptions.
Equal turns ratio, percentage impedance, and resistance to reactance ratio are among the other necessary conditions for paralleling transformers. These conditions ensure balance and stability in the system and guarantee its optimal performance.
Proper use of transformers in parallel is one of the best strategies for increasing efficiency and optimizing energy distribution. These conditions and principles are of paramount importance as guidelines for the successful implementation of a power distribution system.
Eddy currents are an important phenomenon in ferromagnetic materials, describing the occurrence of electric currents in molten cores containing ferromagnetic alloys. In this phenomenon, electric currents circulate as magnetic eddies around various points within the core under the influence of a magnetic field.
Eddy currents in cores are particularly significant in electrical equipment and motors that utilize ferromagnetic materials. This phenomenon can lead to energy losses and voltage drops, necessitating precise design and effective protection in electrical systems.
Finally, this article has reviewed various types of transformers in terms of their structure, applications, and technical features. It briefly covered different types from power transformers in power plants and high-voltage substations to current and voltage transformers used for measurement and protection purposes.
Additionally, topics such as winding connections, paralleling transformers, and the importance of matching conditions in these connections have been discussed. This article aims to provide a comprehensive understanding of these critical devices in electrical systems and offer readers extensive information about transformers.
Read more: Buy ADT iMaster inverter from Iran